LIPOSUCTION TEXTBOOK
The Tumescent Technique By Jeffrey A. Klein MD
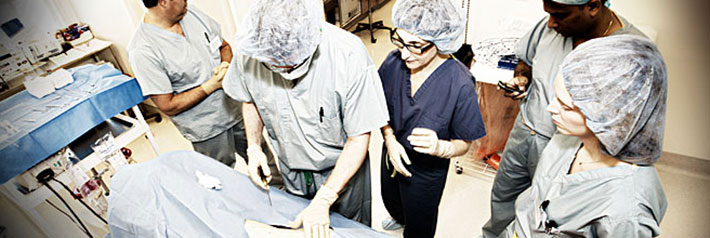
LIPOSUCTION TEXTBOOK
Chapter 17:
Pharmacology of Lidocaine
A review of the basic pharmacology of lidocaine is helpful before attempting to understand the more complex qualities of tumescent lidocaine. Box 17-1 lists physical properties of lidocaine.
Characteristics of Lidocaine
Lipophilia
Lidocaine is a lipophilic molecule that is highly soluble in lipids such as subcutaneous fat. After thoroughly mixing an aqueous solution of lidocaine with an equal volume of the lipophilic solvent octanol, then allowing the mixture to separate by gravity, most of the lidocaine has diffused out of the water and into the octanol. The lidocaine concentration ratio for octanol/water is 43:1, with the water at 25° C (77° F) and buffered to a pH of 7.4. The lidocaine partition coefficient for n-octanol/water (P) is 43/1, and log P is 1.64.
The lipophilic nature of lidocaine accounts for the rapid redistribution of lidocaine into peripheral tissue after an intravenous (IV) injection. The fat/blood concentration ratio at equilibrium is an estimated 1:1 to 2:1.1 Lidocaine lipophilia probably plays a role in the uniquely slow absorption of lidocaine from the tumescent subcutaneous fat into the systemic circulation.
The safety of the relatively large doses of tumescent lidocaine used for tumescent liposuction is the result of a dramatic delay of lidocaine absorption into the systemic circulation. “The big difference in absorption kinetics when highly diluted lidocaine (0.05% [500 mg/L] to 0.10% [1.0 g/L]) is infiltrated subcutaneously arises from lipid binding, buffering lidocaine molecules for delayed release.”2 Since liposuction removes only 20% to 25% of the infiltrated tumescent lidocaine, while liposuction simultaneously removes a much greater percentage of tumescent fat, the lidocaine-lipid binding phenomenon does not entirely explain the unprecedented delay of lidocaine absorption that is so characteristic of tumescent local anesthesia.
In terms of molecular structure, lipophilicity of a local anesthetic is primarily determined by the aromatic group. The addition of carbon atoms to the amide local anesthetic molecule also tends to increase its lipophilia (Figure 17-1).
pKa and pH. The pKa is a constant characteristic of a drug. For lidocaine the pKa is 7.9. Knowledge about lidocaine pKa allows one to generalize about how pH will affect the movement of drugs across a tissue membrane.
According to the pH partition hypothesis, only the nonionized nonpolar form of a drug is sufficiently lipophilic to be able to diffuse across a biologic bilayer lipid membrane. This hypothesis is probably not completely accurate but does provide a qualitative view of what might be happening to lidocaine diffusion at a cellular level. An uncharged lidocaine molecule diffuses across a neuron cell membrane more rapidly than a charged molecule.
The Henderson-Hasselbalch equation for bases states the following:
pH = pKa + log10 (B/BH+)
where B is concentration of nonionized base, and BH+ is concentration of a positively charged ionized base. When a lidocaine solution has a pH of 7.9, then pH = pKa. Therefore:
log10 (B/BH+) = log10 ([Nonion concentration]/[Ion concentration]) = 0
Because log10 (1) = 0, one can conclude that [nonion concentration]/[ion concentration] = 1. Thus, at pH = 7.9, the concentration of the nonionized lidocaine equals that of the ionized form of lidocaine.
The terminal amine group –N–(CH3)2 of lidocaine is a weak base and thus accepts a hydrogen ion, converting the anesthetic into the pharmacologically active, positively charged cationic form. In any solution of lidocaine the proportion of uncharged lidocaine base relative to the positively charged lidocaine cation is a function of the solution’s pH.
The uncharged lidocaine base B is lipophilic and easily diffuses through the lipid bilayer of a cell membrane. The higher the pH of a lidocaine solution, the greater is the proportion of uncharged lidocaine base, and the more rapidly the lidocaine will enter a neuron’s axoplasm. Thus the addition of sodium bicarbonate (NaHCO3) to a solution of lidocaine will promote the entry of lidocaine into neurons. Increased diffusion is manifested clinically by a more rapid onset of anesthesia.
When comparing all amide-type local anesthetics, at any given pH the lower an anesthetic’s pKa, the greater is the proportion of its uncharged base. Also, the more rapid the anesthetic’s diffusion into neurons, the more rapid is the onset of conduction blockage. Knowing that the pKa for lidocaine is 7.9 and the pKa for bupivacaine 8.2, one can predict that lidocaine will have a more rapid onset of anesthesia than bupivacaine.
The positively charged cationic molecule interacts with the neuron’s local anesthetic receptor site. When only the pH of an anesthetic solution bathing mammalian nonmyelinated fibers is varied, a solution with a pH of 7.2 and one with pH of 9.6 will block and unblock the neurons, respectively. Thus, with lidocaine toxicity, metabolic and respiratory acidosis is more dangerous than respiratory alkalosis. Acidosis and hypercapnia increase the central nervous system (CNS) toxicity of lidocaine.3 When treating lidocaine toxicity, therefore, it is imperative to maximize ventilation and oxygen delivery.
Local Anesthetic Solubility
An amine local anesthetic base such as lidocaine is poorly soluble in water and unstable when exposed to air. The lidocaine base is weakly basic and tends to combine with acids to form salts. Depending on the drug’s pKa and the pH of an aqueous solution, the salt of an amine local anesthetic is dissociated and exists in a state of equilibrium between the positively charged quaternary cation and the uncharged lipid-soluble, free-base tertiary amine.
Alkalinization of a amine local anesthetic solution with NaHCO3 shifts the equilibrium toward an increase in the amount of uncharged free base. The rate of diffusion of a local anesthetic is a function of the concentration of its free base, which in turn is a function of the pH of the solution. The uncharged molecule more readily diffuses across the lipid cell membrane and accelerates the onset of local anesthetic action.
Too much alkalinization decreases the amine solubility, however, causing it to precipitate. Alkalinization can decrease the shelf life of an amine local anesthetic and increase the risk of precipitation. After an injection, any precipitation of a local anesthetic solution into tissue can cause injury to the local tissues.
An acidic solution of a local anesthetic has a larger proportion of positively charged quaternary cations, which is less effective because the molecules diffuse much more slowly.
Relative Local Anesthetic Potency. The relative potency of two local anesthetics can be compared by measuring the minimum concentration necessary to block a ceratin nerve.4 Bupivacaine is approximately four times as potent as lidocaine. An alkaline solution of lidocaine, however, might be more effective than an acidic solution of bupivacaine (Case Report 17-1).
pH of Tumescent Solution
Commercially available lidocaine is acidified with hydrochloric acid to protonate the amide nitrogen forming a cation. Nonionized lidocaine molecules, although relatively insoluble in water, are lipid soluble and can more readily cross the lipid cellular wall and enter a neuron.
To optimize the solubility of lidocaine and the stability of epinephrine, commercially available solutions are acidic: pH of 6.5 for lidocaine and pH of 4.5 for lidocaine with epinephrine.
Unfortunately, acidic solutions produce a painful stinging sensation on intradermal or subcutaneous injection. The stinging discomfort of an injection of lidocaine can be attenuated by the addition of NaHCO3 to neutralize the pH of the commercially available preparation.5 Similarly, the use of sodium hydroxide to neutralize the pH of the anesthetic solution also reduces the pain on infiltration.6 Commercially available solutions of lactated Ringer’s solution (LR) do not provide relief from the stinging associated with acidic lidocaine solutions with epinephrine.
When the tumescent technique was originally conceived, it was not known that neutralizing the acid solution by adding NaHCO3 would dramatically attenuate the pain on injection of the anesthetic solution. In the early days of tumescent liposuction the stinging pain on injection of the tumescent solution was so intense that it usually required supplemental intramuscular (IM) meperidine (Demerol) and diazepam (Valium). Adding NaHCO3 to the dilute anesthetic solution eliminated most of the stinging sensation so that meperidine and diazepam could be discontinued. Eliminating narcotics and parenteral sedatives removed the risk of hypoventilation and hypoxemia. Neutralization with NaHCO3 by reducing the need for narcotics is largely responsible for the dramatic safety of tumescent liposuction as an office procedure.
Epinephrine is unstable and will degrade spontaneously in a neutral (pH 7.0 to 7.4) solution, having a half-life of about 10 to 14 days. Because of this instability of epinephrine, tumescent anesthetic solutions for liposuction should be freshly mixed on the day of surgery.
The current formulation of tumescent lidocaine and epinephrine includes 10 mEq NaHCO3/L solution. Sodium bicarbonate is available in several concentrations; for ease of measurement the preferred concentration is the 8.4% solution, which is equivalent to 1 mEq/ml.
Moles, Molar Mass, and Molecular Weight
The current trend in scientific literature is to specify amounts of a drug in terms of moles. The mole is a unit of measurement for mass. A mole (abbreviated mol) of a substance is the number of units of that substance equal to the number of carbon atoms in exactly 12 g of pure12C. This number is well known as Avogadro’s number, 6.022 × 1023. One mole of something consists of exactly 6.022 × 1023 units of that substance. One mole of lidocaine base consists of 6.022 × 1023 molecules of lidocaine.
The molar mass of a substance is the mass in grams of 1 mol of the compound. Although the term molecular weight has been used for this concept, molar mass is scientifically more precise and is preferred in modern chemistry texts.7 One mole of lidocaine base has a molar mass that weighs 234.34 g.
Lidocaine is more soluble in water as an ionic hydrophilic salt. Commercial preparations of aqueous lidocaine use hydrogen chloride as the salt. The molar mass (molecular weight) of lidocaine HCl is 270.8:
1 g lidocaine salt = (1 g)/(270.8 g/mol) = (1/270.8) mol = (0.00369 mol) = 3.69 mmol
In other words, the following inverse relationship exists:
1 g lidocaine HCl = 3.69 mmol
1 mol lidocaine HCl = 270.8 g
Solution Composition: Molarity. A solute is the substance, such as lidocaine, being dissolved. The solvent is the dissolving medium, such as isotonic physiologic saline or LR. Qualitative terms such as dilute (relatively little solute) and concentrated (relatively large amounts of solute) are not sufficiently precise for pharmacologic or chemical calculations.
Modern scientific literature describes solutions in terms of molarity. By definition:
Molarity (M) = (Moles of solute)/(Liters of solvent)
Thus, for lidocaine:
1 M = 1 mol/L = 270.8 mg/L
Commercial preparations of lidocaine intended for subcutaneous injection specify concentration in terms of “grams percent.” A typical commercial preparation is 1% lidocaine = 1 g/100 ml = 1000 mg/100 ml = 10 g/L = 36.9 mM solution. Thus:
1% lidocaine = 36.9 mM solution
The millimolar (mM) solution of a drug is determined as follows: first convert the milligram dose to gram dose by dividing the milligram dose by 1000, and then divide the gram dose by the molar mass (molecular weight) of the drug.
Dilutions: Definitions and Measurements
As noted, commercially available formulations of local anesthetics usually specify lidocaine concentration in “grams per 100 ml” (grams percent). Thus a 1% lidocaine solution contains 1 g of lidocaine per 100 ml of solution, which is equivalent to 10 mg/ml.
Similarly, the traditional specification for epinephrine concentration is defined as a ratio of 1 g of solute to the number of milliliters of solution required to provide the desired concentration. For example, a 1:1000 solution of epinephrine contains 1 g of epinephrine in 1000 ml of solution, or 1 mg per 1 ml. Thus a tumescent solution having an epinephrine concentration at 1:1 million contains 1 g per 1 million ml, or 1 mg/1000 ml.
These traditional designations are sufficient when a surgeon simply needs to specify which of the off-the-shelf local anesthetics is being ordered. When ordering the formulation of customized solutions for tumescent local anesthesia, however, this method of specifying dilutions requires mathematic calculations that are cumbersome and prone to error.
Tumescent Specifications. Formulations of tumescent lidocaine and epinephrine solutions should be specified in terms of milligrams per liter (mg/L). Similarly, the amount of NaHCO3 is specified in terms of milliequivalents per liter (mEq/L).
This method of specifying the formulation of a dilute solution of local anesthesia is easy for staff to use and understand. When multiple bags of anesthetic are used on one patient, this also provides a clear method for keeping tract of the total dose (mg) of lidocaine that has been given.
The task of mixing solutions of tumescent local anesthetic is simplified because a 50-ml bottle of 1% lidocaine contains 500 mg, and therefore two 50-ml bottles contain 1 g of lidocaine. Similarly, a 1-ml ampule of epinephrine at 1:1000 contains 1 mg of epinephrine.
To obtain a tumescent anesthetic solution consisting of 1250 mg of lidocaine and 1 mg of epinephrine per approximately 1 L of isotonic saline for abdominal liposuction, 2.5 bottles (125 ml) of 1% lidocaine and 1 ampule (1 ml) of epinephrine 1:1000 are added to a liter bag of isotonic saline. A compulsively accurate specification of this dilution would show the volume of solution as follows:
1136 ml = 1 L isotonic saline + 125 ml lidocaine + 10 ml NaHCO3 + 1 ml epinephrine
Because no clinically significant difference exists between the effects of 1250 mg of lidocaine in 1136 ml or 1000 ml of solution, there is no compelling need to end up with exactly 1000 ml of solution by first subtracting 136 ml of isotonic saline before adding the solutes.
Plastic bags are preferred over glass bottles, because bags can easily expand and accommodate the extra volumes that are added.
Hyaluronidase Counterproductive
Attempts at vasoconstriction in the earliest days of liposuction used a mixture of undiluted lidocaine, epinephrine, and hyaluronidase, which was injected into the targeted fat after induction of general anesthesia. The rationale for using hyaluronidase was the drug’s alleged ability to augment the diffusion of epinephrine. Although this attribute of hyaluronidase is not well documented, some surgeons still include it in their anesthetic formulations for tumescent liposuction.
Hyaluronidase does not improve the degree of anesthesia achieved by the tumescent technique. It accelerates the rate of absorption of lidocaine8 and produces greater pain on subcutaneous infiltration. Hyaluronidase is a protein and can cause allergic reactions. Therefore it is not recommended for tumescent liposuction.
Effects of Lidocaine
Plasma Protein Binding
Plasma protein binding of lidocaine occurs most importantly to α1–acid glycoprotein, an acute-phase reactant protein (high affinity, low capacity). Lidocaine binding to albumin (low affinity, high capacity) is less important.9 The affinity of lidocaine for protein binding sites can vary with changes in pH and hemodilution.10
The concentration of α1-acid glycoprotein and the concentration of free (unbound) lidocaine can vary widely in the setting of clinical inflammation. Because of variability in serum protein binding with surgery and in certain disease states, one cannot predict free lidocaine simply by knowing the total (free plus protein-bound) lidocaine concentration.11 Surgery, trauma,12 postoperative inflammation,13 cancer, smoking, and myocardial infarction14 all increase α1-acid glycoprotein and lidocaine protein binding, thus decreasing the proportion of free lidocaine. Such patients may tolerate higher doses of lidocaine before encountering toxicity.15 Oral contraceptive agents decrease α1-acid glycoprotein.
The ratio of free lidocaine to total plasma lidocaine levels affects the predictability of lidocaine toxicity based on lidocaine plasma concentrations.16 The proportion of free, unbound lidocaine in plasma increases with increasing concentration. In the clinically therapeutic range of 1 to 4 μg/ml, 20% to 40% of lidocaine is free and not bound to plasma proteins.
Sodium Channel Blocking
Lidocaine inhibits the transmission of nerve impulses by blocking sodium ion (Na+) flux across nerve membranes. Lidocaine blocks nerve membrane Na+ channels in much the same way as calcium channel blockers block Ca++ channels. Blocking the Na+ channels of nerves slows the rate of depolarization sufficiently to prevent the attainment of threshold potential and propagation of an action potential.
In canine papillary muscle, lidocaine displaces cocaine from the Na+ channel receptor through competitive binding.17 Lidocaine may prove to be beneficial in reversing cocaine-induced slowing of ventricular conduction. On the other hand, cocaine and lidocaine are synergistic in producing seizures.18
Nerve Fiber Effects
Local anesthetics tend to block small nerve fibers sooner than larger fibers and nonmyelinated fibers sooner than myelinated fibers. Clinically this is manifested by the observation that small myelinated Aδ fibers that mediate pain and temperature are blocked more easily than the larger myelinated Aα (motor and proprioception), Aβ (proprioception and vibrations), and Aγ (muscle tone) fibers. Patients should not feel pain during tumescent liposuction after good tumescent anesthesia; however, they will often be aware of vibrations and a peculiar rasping sensation during liposuction of deeper planes.
Differential Onset of Neural Blockade. Deposition of the lidocaine very close to a sensory nerve produces immediate local anesthesia. Different types of nerve fibers have different degrees of myelinization and are affected by local anesthesia to a different degree. Autonomic nerve blockade precedes sensory nerve blockade.
Motor nerve activity is the least susceptible to the local effects of lidocaine. Clinical experience suggests that tumescent anesthesia of adipose tissue produces a rapid blockade of pain and temperature sensation, whereas proprioception and vibration sensation remain unaffected.
Neural Blockade as Function of Concentration. In myelinated nerve fibers, at least three consecutive nodes of Ranvier must be blocked before neural impulse propagation is interrupted.19 In vitro the blockade of a nerve seems to be a simple function of the concentration of the local anesthetic; thin individual nerves are readily blocked by a lidocaine concentration of 100 μM.20
In vivo the anesthesia produced by the tumescent technique seems to be a function of two variables: the concentration of dilute lidocaine and the length of nerve exposed to the anesthetic.
Central Nervous System Effects. In experimental animals, lidocaine has significant antiepileptic activity at plasma concentrations of 0.5 mg/L to 4.0 mg/L, the therapeutic range for antiarrhythmic (antidysrhythmic) activity. At plasma levels greater than 7.5 mg/L, however, lidocaine can cause seizures.21 Because the toxic effects of local anesthetics are additive, lidocaine should not be used to treat a seizure or cardiotoxicity caused by another local anesthetic.
Antiinflammatory Effect. Lidocaine inhibits granulocyte adherence and prevents granulocyte delivery to inflammatory sites after an IV infusion of lidocaine in rabbits with aseptic peritonitis.22 In this regard, lidocaine is 10 times more effective than methylprednisolone.
Antibacterial Effects
Tumescent liposuction is associated with a low risk of postoperative infections. This clinical record provides strong evidence that in vivo tumescent local anesthesia is bacteriostatic and bactericidal.
Since 1985 I have documented only one postoperative wound infection among my patients (see Chapter 12). This infection, involving a localized subcutaneous Staphylococcus aureus abscess of the medial thigh, was treated by incision, drainage, and oral antibiotics. Few, if any, routine surgical procedures have a lower incidence of infection than tumescent liposuction.
Among cosmetic surgeons, the exceptionally low infection rate associated with tumescent liposuction is widely regarded as a clinical fact. Other surgical specialists, however, believe that the bactericidal effects of lidocaine are controversial. Consequently, studies have attempted to prove the bactericidal effects of lidocaine by in vitro experimentation.
Reports are conflicting regarding the concentration of lidocaine that is necessary to achieve bactericidal effects in vitro. In part this discrepancy may be explained by the in vitro methodology. The number of bacteria used in the inoculum is an important factor when using in vitro methods to estimate the antimicrobial potency of an antimicrobial agent.23 On the one hand, if an inoculum is too large, an antimicrobial may be judged ineffective because of the high probability of a spontaneous mutation into resistant bacteria.24 On the other hand, if the inocula are too small, too few colonies may be present among the controls to establish a statistically relevant bactericidal effect at 99.9% killing.25,26 The preferred number of bacteria for an in vitro inocula is probably in the range of 105 to 106 bacteria or colony-forming units (CFU) per sample.24,25
One recent in vitro study found that prolonged exposure to 0.1% lidocaine is insufficient to inhibit 108 CFU/ml of various bacteria.27 In contrast, two recent reports have found that dilute lidocaine in a concentration of 0.05% does have in vitro antibacterial activity. One study found this lidocaine dilution to be bacteriostatic for S. aureus.28 The other study used suspensions of bacteria containing approximately 105 CFU/ml. All gram-positive organisms tested, including S. aureus, had significantly lower colony counts in 0.05% or higher concentrations of lidocaine diluted by propofol. All gram-negative organisms had significantly lower colony counts in lidocaine concentrations of 0.2% or higher.29
Some anesthesiologists add 10 mg of lidocaine to 20 ml of propofol, which is equivalent to 0.05% lidocaine, to decrease the pain on IV injection of propofol.30 Higher lidocaine concentrations may be incompatible with the stability of propofol in emulsion.31
Lidocaine’s antibacterial activity, in addition to its local anesthetic properties, has not been fully appreciated.32 When first recognized as being bacteriostatic, clinicians were concerned that lidocaine might inhibit bacterial growth in cultures of tissue obtained by biopsy using local anesthesia.33-39 In vitro evidence indicates that lidocaine is not only bacteriostatic but actually bactericidal for organisms isolated from skin lesions.40 Recently it has been shown that adding NaHCO3 to lidocaine augments this in vitro bactericidal activity.41
Lidocaine is antibacterial for both gram-negative and gram-positive bacteria. In gram-negative bacteria such as Escherichia coli, Salmonella typhimurium, and Pseudomonas aeruginosa, lidocaine appears to act synergistically with antibiotics by depolarizing the bacterial cell membrane and increasing cell membrane permeability.42
Bupivacaine, which is structurally related to lidocaine, also has antibacterial properties. A mixture of racemic bupivacaine appears to have more in vitro antibacterial activity than levobupivacaine.43
From the point of view of dermatologic surgery, the significance of the bactericidal activity of lidocaine is that this local anesthetic agent might be responsible for preventing wound infections. Although no in vivo clinical studies have substantiated this presumed effect, this should not be ignored. When a surgical procedure is performed on skin using general anesthesia, concomitant use of lidocaine might be warranted.
The minimal incidence of postoperative infections associated with tumescent liposuction may be attributed to the following clinical factors:
- In vivo bacteriostatic and bactericidal effects of lidocaine combined with epinephrine and bicarbonate
- Low incidence of hematomas and seromas associated with tumescent liposuction
- Moderation in terms of the surgical trauma inflicted during a single surgical procedure
- Judicious selection of healthy patients
The last two factors are of critical importance. Common sense and moderation in liposuction are crucial to reduce postoperative complications, including infections.
Neurotoxicity
Local anesthetics, including lidocaine, can produce a concentration-dependent chemical toxicity to nerve tissue. Lidocaine induces a nonreversible loss of impulse activity in frog nerve in a progressive fashion with increasing drug concentration, beginning at a concentration of 1% (40 mM).44
Although the range of lidocaine concentration that produces toxicity in mammalian nerves is not known, clearly the least effective concentration is the safest.
References
- Rosenberg PH, Kyttä J, Alila A: Absorption of bupivacaine, etidocaine, lignocaine and ropivacaine into n-heptane, rat sciatic nerve, and human extradural and subcutaneous fat, Br J Anaesth 58:310-314, 1986.
- de Jong R: Personal communication, 1999.
- McCaughey W: Adverse effects of local anesthetics, Drug Safety 7:178-189, 1992.
- Strichartz GR, Berde CB: Local anesthetics. In Miller RD, editor: Anesthesia, ed 4, New York, 1994, Churchill Livingstone.
- Mckay W, Morris R, Mushlin P: Sodium bicarbonate attenuates pain on skin infiltration with lidocaine, with or without epinephrine, Anesth Analg 66:572-574, 1987.
- Stewart JH, Chen SE, Cole GW, Klein JA: Neutralized lidocaine with epinephrine for local anesthesia. II. J Dermatol Surg Oncol 16:842-845, 1990.
- Zumdahl SS: Chemistry, ed 3, Lexington, Mass, 1993, Heath.
- Nathan N, Benrhaiem M, Lotfi H, et al: The role of hyaluronidase on lidocaine and bupivacaine pharmacokinetics after peribulbar blockade, Anesth Analg 82:1060-1064. 1996.
- Routledge PA, Barchowsky A, Bjornsson TD, et al: Lidocaine plasma protein binding, Clin Pharmacol Ther 27:347, 1980.
- Remmel RP, Copa AK, Angaran DM: The effects of hemodilution, pH, and protamine on lidocaine protein binding and red blood-cell uptake in vitro, Pharm Res 8:127, 1990.
- Landow L, Wilson J, Heard SO, et al: Free and total lidocaine levels in cardiac surgical patients, J Cardiothorac Anesth 4:340-347, 1990.
- Slaughter RL, Hassett JM: Hepatic drug clearance following traumatic injury, Drug Intell Clin Pharm 19:799-806, 1985.
- Wood M: Plasma drug binding: implications for anesthesiologists, Anesth Analg 65:786, 1986.
- Thomson AH, Kelman AW, de Vane PJ, et al: Changes in lignocaine disposition during long-term infusion in patients with acute ventricular arrhythmias, Ther Drug Monit 9:283-291, 1987.
- Kessler KM, Kissane B, Cassidy J, et al: Dynamic variability of binding of antiarrhythmic during the evolution of acute myocardial infarction, Circulation 70:472-478, 1984.
- Pieper JA, Wyman MG, Goldreyer BN, et al: Lidocaine toxicity: effect of total versus free lidocaine concentrations, Circulation 62(suppl III):181, 1980.
- Liu D, Hariman RJ, Bauman JL: Cocaine concentrationeffect relationship in the presence and absence of lidocaine: evidence of competitive binding between cocaine and lidocaine, J Pharmacol Exp Ther 276:568-577, 1996.
- Barat SA, Abel-Rahman MS: Cocaine and lidocaine in combination are synergistic convulsants, Brain Res 742:157-162, 1996.
- Tasaki I: Nervous transmission, Springfield, Ill, 1953, Thomas.
- Rosenberg PH, Heavner JE: Temperature dependent nerve blocking action of lidocaine and halothane, Acta Anaesthesiol Scand 224:314, 1980.
- Julien RM: Lidocaine in experimental epilepsy: correlation of anticonvulsant effect with blood concentrations, Electroencephalogr Clin Neurophysiol 34:639, 1973.
- MacGregor RR, Thorner RE, Wright DM: Lidocaine inhibits granulocyte adherence and prevents granulocyte delivery to inflammatory sites, Blood 56:203, 1980.
- Wolfson JS, Swartz MN: Drug therapy: serum bactericidal activity as a monitor of antibiotic therapy, N Engl J Med 312: 968-975, 1985.
- Pearson RD, Steigbigel RT, Davis HT, Chapman SW: Method of reliable determination of minimal lethal antibiotic concentrations, Antimicrob Agents Chemother 18:699-708, 1980.
- Reller LB, Stratton CW: Serum dilution test for bactericidal activity. II. Standardization and correlation with antimicrobial assays and susceptibility tests, J Infect Dis 136:196-204, 1977.
- Morello JA, Mizer HE, Wilson ME, Granato PA: Tools and techniques in microbiology. In Johnson M, editor: Microbiology in patient care, Dubuque, Iowa, 1994, Brown.
- Craig SB, Concannon MJ, McDonald GA, Puckett CL: The antibacterial effects of tumescent liposuction fluid, Plast Reconstr Surg 103:666-670, 1999.
- Kirk GA, Koontz FP, Chavez AJ: Lidocaine inhibits growth of Staphylococcus aureus in propofol, Anesthesiology 77:A407, 1992.
- Gajraj RJ, Hodson MJ, Gillespie JA, et al: Antibacterial activity of lidocaine in mixtures with Diprivan, Br J Anaesth 81:444-448, 1998.
- Gehan G, Karoubi P, Quinet F, et al: Optimal dose of lignocaine for preventing pain on injection of propofol, Br J Anaesth 66:324-326, 1991.
- Lilley EMM, Isert PR, Carasso ML, Kennedy RA: The effect of the addition of lignocaine on propofol emulsion stability, Anaesthesia 51:815-818, 1996.
- Murphy JT, Allen HF, Mangiaracine AB: Preparation, sterilization, and preservation of ophthalmic solutions, Arch Ophthalmol 53:63-78, 1955.
- Schmidt RM, Rosenkranz HS: Antimicrobial activity of local anesthetics: lidocaine and procaine, J Infect Dis 121:597-607, 1970.
- Ravin CE, Latimer JM, Matsen JM: In vitro effects of lidocaine on anaerobic respiratory pathogens and strains of Haemophilus influenzae, Chest 72:439-441, 1977.
- Wimberley N, Willey S, Sullivan N, Bartlett JG: Antibacterial properties of lidocaine, Chest 76:37-40, 1979.
- Sculley PD, Dunley RE: Antimicrobial activity of a lidocaine preparation, Anesth Prog 27:21-23, 1980.
- Badenoch PR, Coster DJ: Antimicrobial activity of topical anaesthetic preparations, Br J Ophthalmol 66:634-667, 1982.
- Morrow ME, Berry CW: Antimicrobial properties of topical anesthetic liquids containing benzocaine, Anesth Prog 35:9-13, 1988.
- Czinn SJ, Carr HS, Speck WT: Effects of topical anesthetic agents on Campylobacter pylori, J Pediatr Gastroenterol Nutr 9:46-48, 1989.
- Miller MA, Shell WB: Antimicrobial properties of lidocaine on bacteria isolated from dermal lesions, Arch Dermatol 121: 1157-1159, 1985.
- Thompson KD, Welykyj S, Massa MC: Antibacterial activity of lidocaine in combination with a bicarbonate buffer, J Dermatol Surg Oncol 19:216-220, 1993.
- Ohsuka S, Ohta M, Masuda K, et al: Lidocaine hydrochloride and acetylsalicylate kill bacteria by disrupting the bacterial membrane potential in different ways, Microbiol Immunol 38:429-434, 1994.
- Hodson M, Gajraj R, Scott NB: A comparison of the antibacterial activity of levobupivacaine vs. bupivacaine: an in vitro study with bacteria implicated in epidural infection, Anaesthesia 54:699-702, 1999.
- Bainton CR, Strichartz GR: Concentration dependence of lidocaine-induced irreversible conduction loss in frog nerve, Anesthesiology 81:657-667, 1994.
Figure 17-1 Lidocaine is representative of amide local anesthetics. Molecule has lipophilic aromatic fraction, which facilitates absorption into adipose tissue. Hydrophilic amino terminal portion of lidocaine molecule facilitates solubility in water as a salt of hydrochloride.
CASE REPORT 17-1 Bupivacaine Versus Lidocaine |
To decrease the amount of pain on injection, it is common practice to neutralize a commercial solution of lidocaine (pH 4 to 6) by adding a small amount of sodium bicarbonate (NaHCO3). By analogy a surgeon added NaHCO3 to a solution of bupivacaine. When a precipitate formed within the bottle of bupivacaine, the solution was discarded. Despite noting a precipitation on a second attempt to mix a neutralized solution of bupivacaine, the surgeon injected the solution into the glabella. The result was deep tissue necrosis and a permanent hypopigmented scar. |
Discussion. Bupivacaine is more lipid soluble and less water soluble than lidocaine. Therefore bupivacaine will precipitate more readily than lidocaine on neutralization with the addition of NaHCO3. This is an example of why lidocaine is safer than bupivacaine. |
BOX 17-1 Physical Properties of Lidocaine |
Molecular weight of lidocaine base: MW (base) = 234.34 |
Molecular weight of lidocaine HCl salt: MW (HCl salt) = 270 |
1 mol lidocaine HCl = 270.8 g |
1 g lidocaine HCl = 3.69 mmol (millimoles) |
1 mM (millimolar) = 270.8 mg/L |
Lidocaine pKa = 7.90 |
Partition coefficient (P) for n-octanol/water = 43/1; log P = 1.98; potency = 2.0 |